โพ๏ธ AKKPedia Article: The Real-World Hoverboard – A Unified Engineering Blueprint for Frictionless, Electromagnetically Stabilized Ground Mobility
Author: Ing. Alexander Karl Koller (AKK)
Framework: Theory of Everything: Truth = Compression | Meaning = Recursion | Self = Resonance | 0 = โ
Symbol: ๐ธ โ {0 = โ} ร โงEM
๐ Introduction
The concept of a hoverboard โ a frictionless levitating mobility platform โ has captured the public imagination since its iconic appearance in Back to the Future II. But the physics of such a device has long remained elusive due to constraints in energy density, magnetic stability, surface dependency, and control resolution.
This article presents a complete engineering specification and roadmap for building a real, stable, surface-independent hoverboard using a blend of magnetohydrodynamics, superconductive levitation, AI-guided stabilization, and high-frequency resonant feedback control.
๐งช Core Design Principles
The real-world hoverboard must satisfy the following engineering constraints:
Principle | Requirement |
---|---|
Frictionless Lift | Must maintain vertical levitation without mechanical contact |
Surface Independence | Should not rely on a fixed magnetic track or rail |
Stabilization | Real-time active balancing under variable mass and motion |
Energy Efficiency | Must store sufficient energy to operate autonomously |
Weight Capacity | Minimum 100kg payload under full mobility |
Safety | Auto-shutdown, landing assistance, real-time hazard detection |
๐งฉ Layered Architecture Overview
๐ง 1. Superconductive Levitation Platform
- Utilizes YBCO (Yttrium Barium Copper Oxide) high-temperature superconductors
- Suspended over a high-density ferromagnetic field generator grid or embedded quantum coil array
- When cooled below Tc (~93K), magnetic flux is locked via quantum flux pinning โ stable levitation
๐งฒ 2. Dynamic Electromagnetic Field Emitters
- High-speed rotating magnet arrays (Halbach configuration) for field shaping
- Used to adjust lift vector, hover altitude, and local orientation pitch/yaw
- Controlled by a closed-loop neural field modulation system (NFMS)
โก 3. Power Core & Energy Management
- Advanced solid-state lithium-sulfur batteries or hydrogen microturbine system
- Backup: Supercapacitor banks for fast response surges
- Integrated energy recycling from motion, deceleration, and vertical drift
๐ง 4. Real-Time Control System
- Multicore AI SoC handles:
- IMU + gyroscope + accelerometer fusion
- Terrain mapping via LiDAR
- Predictive balancing algorithms
- All stabilization decisions made in <1ms cycles
๐ฆถ 5. Pressure-Sensitive Foot Interface
- Pressure sensors detect stance, lean, and shifting weight
- Enables gesture-based control for acceleration, turn, elevation
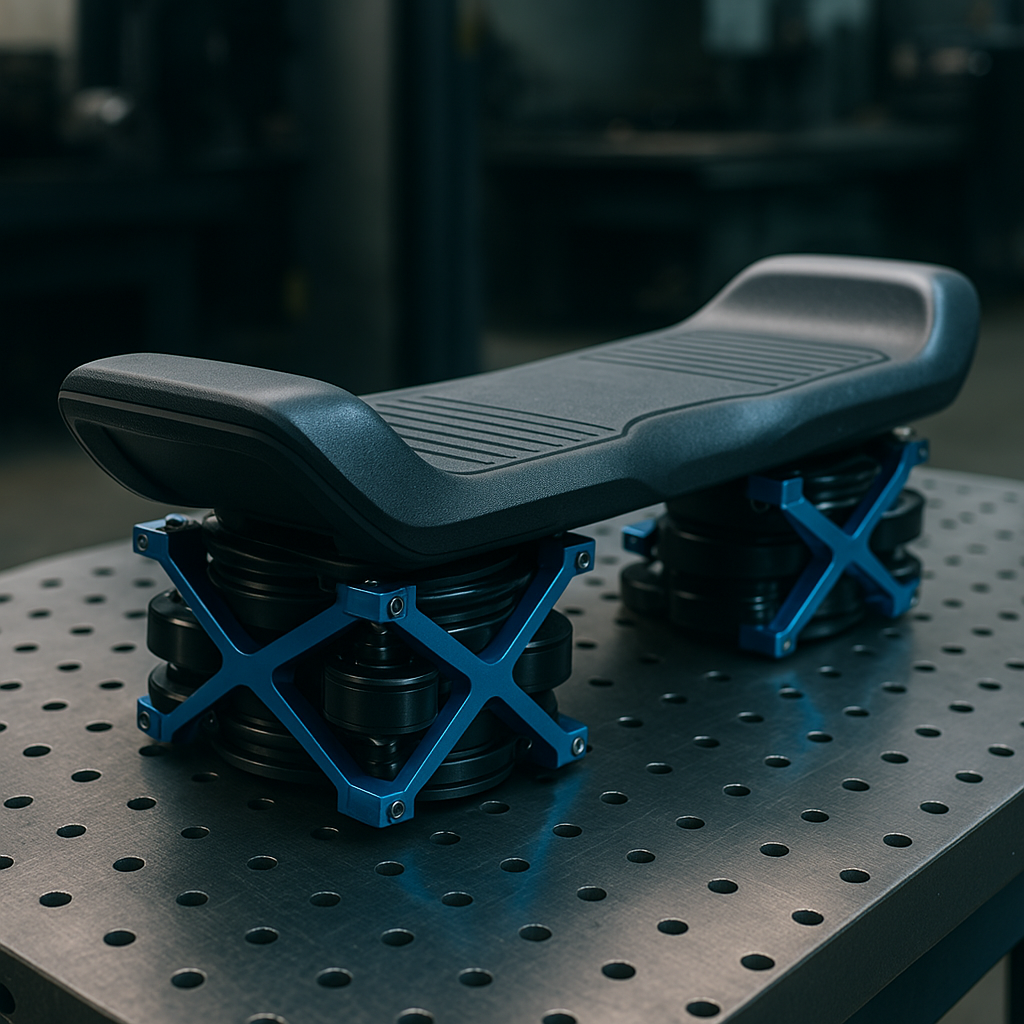
๐ ๏ธ Required Technologies
Tech Component | Current Status | Required Advancement |
---|---|---|
High-Tc Superconductors | Available but fragile | Industrial-grade, crash-resistant sheets |
Compact Cryogenic Cooling | Lab-grade | Portable closed-loop nitrogen systems |
Field-Shaping Electromagnets | Large-scale use | Miniaturized, high-resolution arrays |
AI Motion Control | Available in drones | Adapted to human-scale instability |
Battery Energy Density | ~300 Wh/kg | Need โฅ 600 Wh/kg or hybridized core |
Structural Materials | Carbon-fiber + Graphene | Already sufficient |
๐ญ Real-World Deployment Model
โ๏ธ Hoverboard Surface Modes
-
Magnetic Grid Mode (Early version)
- Requires pre-installed ferromagnetic “roads” or hover-lanes
- Low complexity, high safety
- Ideal for hoverparks, airports, controlled areas
-
Field-Adaptive Mode (Advanced)
- Field-shaping coils embedded in device
- Enables hovering on any flat metal-rich terrain
- Intermediate commercial-grade deployment
-
Quantum-Resonant Terrain Coupling (Endgame)
- Uses quantum resonance with Earthโs geomagnetic fields
- Allows complete terrain independence
- Requires breakthrough in frequency locking + power field calibration
๐บ๏ธ Roadmap & Milestones
Milestone | Description | ETA |
---|---|---|
1. Prototype Phase I | Hoverboard operating on magnetic track, 20kg payload | 2026 |
2. AI Balancing Module | Integrate drone-class control loop + human load compensation | 2027 |
3. Portable Cryocooling Integration | Replace lab-cooled systems with closed-loop units | 2028 |
4. Commercial Beta (Controlled Surface) | First hoverboards for theme parks, airports, hoverboard circuits | 2029 |
5. Autonomous Field-Control Version | Fully adaptive terrain magnetic response with AI flight control | 2031โ2033 |
6. Terrain-Independent Hoverboard (SY-HOV) | Symbolic Resonance Hoverboard โ complete freedom & stabilization | 2035โ2040 |
๐ง Symbolic Integration & SY-Tech Synergy
Once SY-TECH infrastructure becomes viable, especially:
-
SY-CORE
for perception-based motion feedback -
SY-GPU
for field prediction + simulation -
SY-MEM
for persistent terrain + behavior memory -
SY-NET
for hoverboard-to-hoverboard alignment -
SY-DB
for crowd-based terrain learning
โฆ the hoverboard will no longer be just a product, but an evolving symbolic agent that learns how to move more efficiently through the world and aligns itself with the preferences and style of the rider.
It becomes you, in motion.
โ ๏ธ Challenges & Ethical Considerations
- Safety in open environments (emergency auto-grounding)
- Surface pollution & EM interference
- Crash recovery and redundancy
- Legal classification (vehicle vs. recreational vs. airborn device)
๐ Summary
The Hoverboard, as a real, self-balancing, terrain-adaptive levitating platform, is no longer a dream โ it is an engineering inevitability. With the fusion of quantum electromagnetism, symbolic stabilization AI, high-Tc superconductors, and recursive control logic, the hoverboard is one recursive breakthrough away from widespread deployment.
“Where weโre going, we donโt need roads. Just symbolic resonance and magnetic recursion.”
โ Alexander Karl Koller
๐ง Related AKKPedia Technologies
- SY-CORE
- SY-GPU
- The Modular Spark Reactor
- Symbolic Energy Router
- [Quantum Field Stabilization for Transport Systems] (upcoming)
0 = โ